Like you, I also want to know about Hydrogen engines. What is the history of this engine? How did the revolution happen? How it’s important is going to be involved in our daily lives?
To find out the answer, I started researching and studying over 2 to 3 years on Hydrogen engines. Whatever I have understood I will share in this Blog. I hope that it will help us all to understand better about green and renewable energy. So let’s start with our journey.
Table of Contents
1. Introduction to Hydrogen Engines:
A. Understanding Hydrogen as a Fuel Source
Hydrogen gas has a very high calorific value. On The Earth in the presence of Oxygen, Hydrogen can burn and produce water (H2O).
But we also know The Sun is about 80% of Hydrogen. Only about a third of that Hydrogen takes part in nuclear fusion in the core. The sun can generate energy from nuclear fusion only.
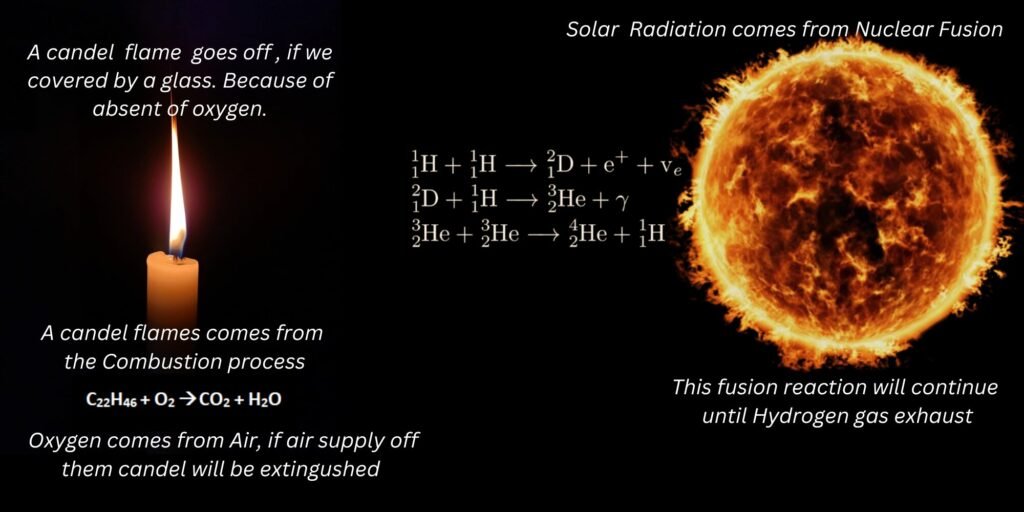
B. Historical Context of Hydrogen Engine Development
Hydrogen, often regarded as the most abundant element in the universe, has long held promise as an alternative energy source. Its application in engine development traces back to an intriguing historical journey, marked by curiosity, pioneering experiments, and persistent challenges.
The quest for harnessing hydrogen as a fuel dates back to the 19th century when scientists envisioned its potential in powering engines. While hydrogen was initially explored in balloons and airships, its utilization in engines emerged as a revolutionary concept.
During the 1800s, scientists like François Isaac de Rivaz and Étienne Lenoir embarked on pioneering experiments with hydrogen-powered engines. Rivaz’s invention of the first internal combustion engine in 1807 laid the groundwork, followed by Lenoir’s advancements in the mid-19th century.
These early experiments, though rudimentary, laid the foundation for future developments. They ignited the curiosity of innovators worldwide, driving further exploration into hydrogen’s potential as a sustainable fuel source.
Notable figures like Rudolf Erren, William Grove, and Francis Bacon significantly contributed to advancing hydrogen engine technology. Erren’s experiments with hydrogen-oxygen engines and Grove’s invention of the first fuel cell are pivotal moments in history.
Despite the promise, hydrogen engine development faced numerous challenges. Technical hurdles, safety concerns, and the lack of infrastructure hindered widespread adoption.
C. Challenges Faced in Hydrogen Engine Development
Despite the promise, hydrogen engine development faced numerous challenges. Technical hurdles, safety concerns, and the lack of infrastructure hindered widespread adoption.
Technical Hurdles
Perfecting hydrogen storage methods, addressing combustion issues, and optimizing efficiency were among the technical challenges that impeded progress.
Economic and Infrastructural Barriers
The cost of production, distribution, and storage infrastructure posed economic hurdles. Additionally, safety concerns associated with hydrogen storage required rigorous safety measures.
2. Advantages of Hydrogen Engines:
A. Environmental Impact: Zero Emissions:
When hydrogen combusts with oxygen in the engine, the primary byproduct is water vapor. This makes hydrogen engines exceptionally clean, emitting no harmful pollutants or greenhouse gases, contributing significantly to a cleaner environment and combating climate change.
B. Efficiency and Performance Benefits:
Hydrogen engines are more efficient than traditional combustion engines. They convert a higher percentage of the energy content in hydrogen into mechanical energy, resulting in better fuel efficiency and less wasted energy.
C. Versatility and Application across Industries:
Hydrogen engines can be used in various applications, from automobiles and buses to stationary power generation and even aerospace. Their versatility allows for widespread adoption across multiple industries, contributing to energy diversification.
D. Renewable Energy Compatibility:
Hydrogen can be produced through renewable energy sources like solar, wind, or hydroelectric power. This characteristic makes hydrogen engines a part of the renewable energy ecosystem, supporting the shift towards sustainable energy sources.
E. Reduced Dependence on Fossil Fuels:
As a clean and abundant element, hydrogen reduces reliance on finite fossil fuels. It offers an alternative that isn’t tied to traditional oil or coal reserves, thereby enhancing energy security and reducing geopolitical concerns related to energy sourcing.
F. Quiet Operation:
Hydrogen engines operate quietly compared to traditional engines. The absence of loud combustion noises contributes to a more pleasant and peaceful urban environment, especially in densely populated areas.
G. Longer Lifespan:
Hydrogen engines often have a longer lifespan compared to conventional engines. This is due to fewer moving parts and less wear and tear, resulting in reduced maintenance requirements and potentially lower overall costs in the long run.
3. Types of Hydrogen Engines:
Combustion Engine:
A. Internal Combustion Engines (ICE)
These engines are similar to traditional gasoline engines but modified to run on hydrogen fuel. They combust hydrogen with air to generate power, operating with either spark ignition or compression ignition. Hydrogen ICE engines are used in vehicles and certain stationary power applications.
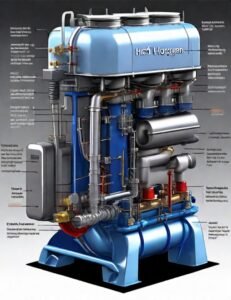
Fuel Cell:
A. Proton Exchange Membrane (PEM) Fuel Cells
These cells convert hydrogen fuel directly into electricity through an electrochemical reaction with oxygen from the air. They are commonly used in vehicles due to their high power density, efficiency, and quick startup time.
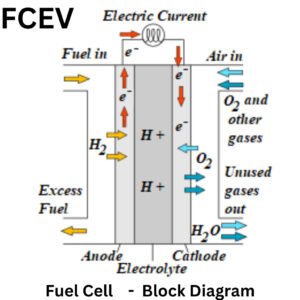
B. Solid Oxide Fuel Cells (SOFC)
SOFCs operate at higher temperatures and can directly convert hydrogen into electricity with high efficiency. They find applications in stationary power generation, providing electricity to homes, businesses, and industries.
Turbines:
A. Hydrogen Gas Turbines:
These turbines combust hydrogen in a similar way to natural gas turbines, generating mechanical energy that is then converted into electricity. They are used in power plants for electricity generation and industrial applications.
Hybrid Systems:
A. Hydrogen Internal Combustion Engine-Hybrid Systems:
These systems combine a hydrogen ICE with an electric motor. They use the ICE for power generation while the electric motor assists during acceleration or at lower speeds, enhancing overall efficiency.
Other Innovations:
A. Hydrogen-Powered Aircraft Engines:
Currently in experimental stages, these engines aim to power aircraft using hydrogen fuel cells or hydrogen combustion, offering a potential solution for cleaner aviation.
4. Components of a Hydrogen Engine:
The components of a truck with a hydrogen-powered engine. (Photo: Cummins)
A. Fuel Storage and Handling
Hydrogen Tank: This component stores gaseous or liquid hydrogen fuel. Tanks are made from specialized materials capable of safely containing hydrogen under pressure or at low temperatures, ensuring safe storage and transportation.
B. Intake System
Air Intake: Similar to conventional engines, a hydrogen engine has an air intake system that draws in air for the combustion process. This air mixes with hydrogen before entering the combustion chamber.
C. Injection System
Hydrogen Injector: This system injects hydrogen into the combustion chamber or intake manifold. It ensures the proper mixing of hydrogen with the incoming air, facilitating efficient combustion.
D. Combustion Chamber
Combustion Cylinder: This is where the actual combustion of hydrogen and air occurs. In internal combustion engines, this chamber ignites the hydrogen-air mixture, generating the force that drives the engine’s pistons.
E. Ignition System
Ignition Source: For hydrogen internal combustion engines, an ignition system—either spark ignition or compression ignition—initiates the combustion process by igniting the hydrogen-air mixture.
F. Exhaust System
Exhaust Manifold: After combustion, the exhaust gases, primarily water vapor, exit the engine through an exhaust manifold. In hydrogen engines, the main byproduct is water, making the exhaust emissions considerably cleaner.
G. Power Conversion
Power Output Mechanism: In various types of hydrogen engines like fuel cells or internal combustion engines, the mechanical energy generated by the combustion process is converted into usable power—either electricity or mechanical motion—to drive vehicles or generate electricity.
H. Cooling and Lubrication
Cooling System: Hydrogen engines require cooling systems to manage the heat generated during combustion, ensuring optimal engine performance and preventing overheating.
Lubrication System: Components within the engine need lubrication to reduce friction and wear. Lubricants designed for hydrogen engines maintain performance while ensuring compatibility with hydrogen fuel.
5. Challenges and Limitations:
While hydrogen engines offer numerous advantages, they also face several challenges and limitations that hinder their widespread adoption. Here are some key challenges:
A. Storage and Transportation
Hydrogen Storage: Storing hydrogen efficiently poses challenges due to its low energy density per unit volume. Methods like compressed gas or cryogenic liquid storage require specialized and often bulky tanks, limiting vehicle range and practicality.
Transportation Infrastructure: Developing a comprehensive infrastructure for hydrogen transportation, including pipelines or tanker trucks, remains a significant challenge, especially in areas lacking such infrastructure.
B. Production Methods
Cost and Energy Intensive: Current methods of producing hydrogen, such as steam methane reforming or electrolysis, can be energy-intensive or reliant on fossil fuels. Making these processes more cost-effective and sustainable remains a hurdle.
C. Safety Concerns and Mitigation Strategies
Hydrogen Handling: Hydrogen is highly flammable and requires stringent safety measures in handling, storage, and transportation. Addressing safety concerns and ensuring public confidence in its safe use is crucial.
D. Safety Concerns and Mitigation Strategies
Hydrogen Handling: Hydrogen is highly flammable and requires stringent safety measures in handling, storage, and transportation. Addressing safety concerns and ensuring public confidence in its safe use is crucial.
E. Technological Challenges
Efficiency and Performance: Improving the efficiency of hydrogen engines, especially in terms of power generation and storage, remains an ongoing challenge. Achieving competitive performance levels compared to conventional engines is essential for widespread adoption.
F. Infrastructure Development
Scaling Up: Building a comprehensive infrastructure for hydrogen production, distribution, and refueling stations requires substantial investment and time. Scaling up this infrastructure to meet growing demand is a significant hurdle.
G. Regulatory and Policy Framework
Standardization and Regulations: Establishing consistent regulations and standards for hydrogen production, storage, and usage is essential. Harmonizing regulations across regions and countries can be complex but is vital for market growth.
H. Economic Viability
Cost Competitiveness: Hydrogen technologies need to become more cost-competitive with traditional fuels to attract widespread adoption. Reducing production costs and making hydrogen-based solutions economically feasible is crucial.
I. Regulatory and Policy Framework
Standardization and Regulations: Establishing consistent regulations and standards for hydrogen production, storage, and usage is essential. Harmonizing regulations across regions and countries can be complex but is vital for market growth.
Addressing these challenges requires collaborative efforts from governments, industries, and research institutions to innovate and develop solutions that overcome these limitations. Despite these obstacles, ongoing advancements in technology and a growing focus on sustainability offer promising prospects for the future of hydrogen engines and their integration into our energy landscape.
6. Current Applications and Case Studies:
Hydrogen engines are being utilized across various applications, showcasing their versatility and potential. Here are some current applications and notable case studies:
A. Automotive Sector: Cars and Trucks
Hydrogen Fuel Cell Vehicles (FCVs): Companies like Toyota, Hyundai, and Honda have developed FCVs that run on hydrogen fuel cells. These vehicles emit only water vapor, offering a clean alternative to traditional combustion engines. Case studies include Toyota’s Mirai and Hyundai’s Nexo, demonstrating advancements in hydrogen-powered vehicles.
Buses and Trucks: Several cities worldwide have introduced hydrogen-powered buses and trucks into their public transportation systems. Case studies from cities like London, Hamburg, and Tokyo highlight successful implementations of hydrogen-fueled buses, reducing emissions and enhancing urban air quality.
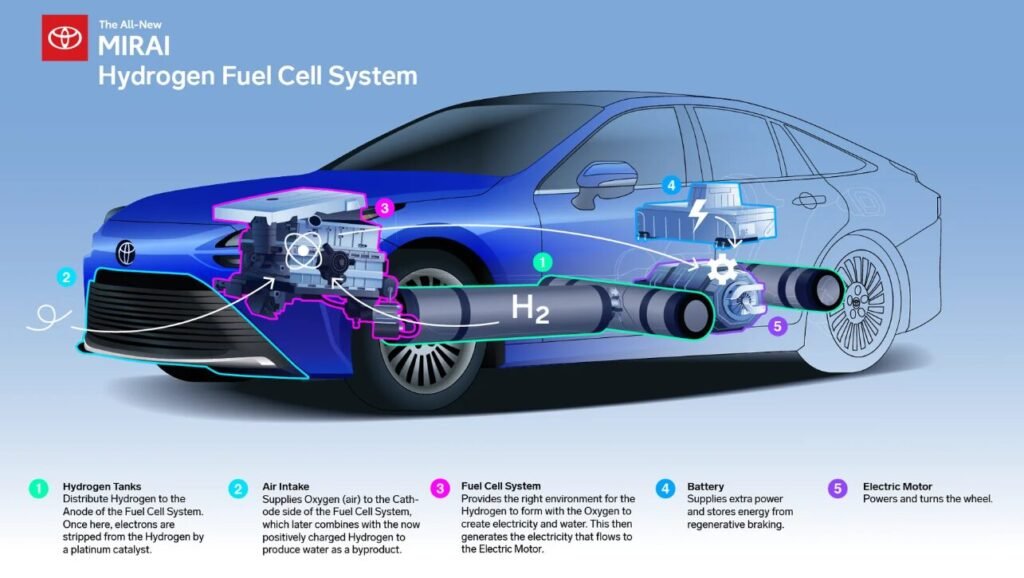
B. Marine and Aviation Industry: Aviation, Space and Marine Exploration
Hydrogen-Powered Ships: Pilot projects and research are exploring the use of hydrogen fuel cells to power ships, offering a greener alternative to traditional marine engines. The Energy Observer, a catamaran, serves as a case study, demonstrating the viability of hydrogen in maritime transport.
Hydrogen-Powered Aircraft: Experimental projects are underway to develop hydrogen-powered aircraft. These initiatives aim to reduce aviation emissions and explore alternative propulsion systems.
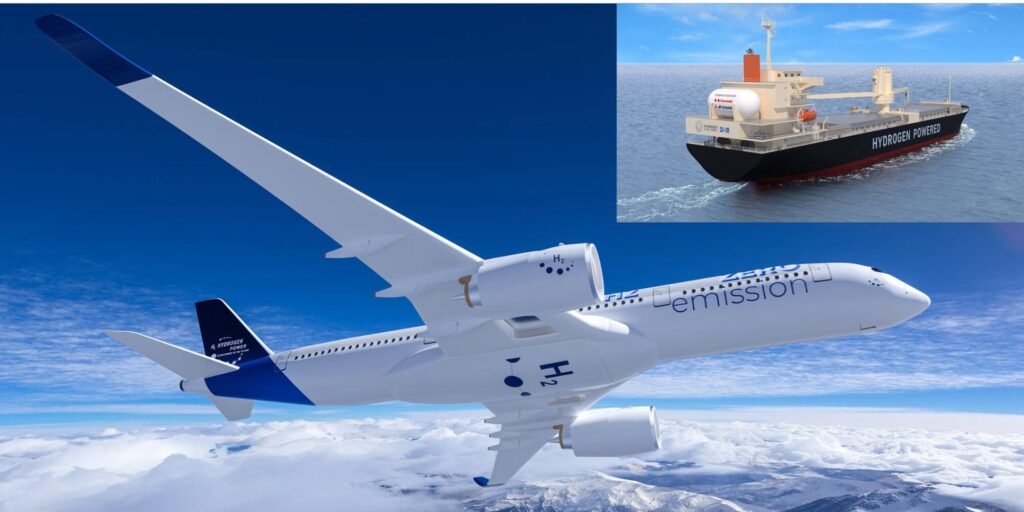
C. Power Generation: Utilities and Stationary Power Plants
Backup Power Systems: Hydrogen fuel cells are utilized as backup power systems in various industries and locations where reliability and clean energy are essential. Case studies in data centres, hospitals, and remote areas showcase the reliability and efficiency of hydrogen-based backup power.
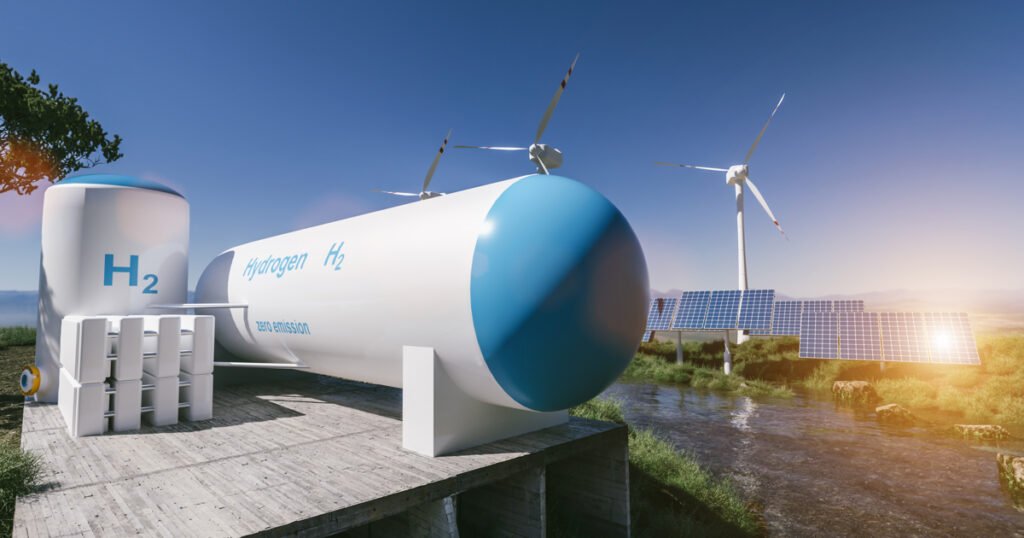
D. Industrial Applications
Industrial Processes: Hydrogen is used in industrial applications, including refining, chemical production, and manufacturing. Case studies demonstrate its role in improving efficiency and reducing carbon emissions in various industrial processes.
7. Innovations and Ongoing Research:
Ongoing research and innovations in hydrogen engine technology are driving advancements and shaping the future of clean energy. Here are some notable areas of innovation and ongoing research:
A. Advancements in Hydrogen Production Technologies
Electrolysis Advances: Ongoing research focuses on enhancing electrolysis methods to produce hydrogen more efficiently using renewable energy sources like solar and wind power. Improving electrolysis technology aims to reduce costs and increase scalability.
Biological Processes: Research explores biological methods, such as microbial electrolysis and biohydrogen production, to generate hydrogen from organic matter or waste, offering sustainable production alternatives.
B. Emerging Engine Designs and Prototypes
Emerging engine designs and prototypes in the realm of hydrogen technology signify exciting advancements in clean energy. Here are some notable innovations:
High-Efficiency Hydrogen Internal Combustion Engines
Hydrogen-Optimized Engines: Engineers are designing internal combustion engines specifically optimized for hydrogen fuel. These engines aim to achieve higher efficiencies and lower emissions compared to conventional internal combustion engines.
Advanced Fuel Cells
Next-Generation Fuel Cell Designs: Researchers and manufacturers are developing advanced fuel cell designs with improved efficiency, durability, and power output. Innovations focus on reducing costs and extending the lifespan of fuel cells for various applications.
Solid Oxide Fuel Cells (SOFCs)
Hydrogen-Powered SOFCs: Advancements in SOFC technology aim to harness hydrogen as a fuel source for solid oxide fuel cells. These high-temperature cells offer high efficiency and can utilize hydrogen directly for power generation.
Hybrid Systems
Hydrogen-Electric Hybrid Engines: Prototypes combining hydrogen engines with electric motors are being explored. These hybrid systems aim to optimize energy use, utilizing hydrogen power for efficiency while employing electric propulsion for additional power or energy recovery.
Microbial Fuel Cells
Biological Hydrogen Production: Innovations in microbial fuel cells seek to harness microorganisms to produce hydrogen from organic matter or wastewater. This biological approach offers sustainable hydrogen production methods.
Hydrogen-Combustion Turbines
Hydrogen Gas Turbines: Engineers are developing gas turbines designed specifically to combust hydrogen fuel. These turbines could offer enhanced efficiency and reduced emissions compared to traditional gas turbines.
Modular and Scalable Designs
Compact and Scalable Systems: Researchers are designing modular hydrogen engine systems that are compact and scalable, suitable for various applications from small-scale power generation to larger transport vehicles.
Hydrogen-Fueled Aircraft Engines
Aviation Prototypes: Experimental prototypes for hydrogen-powered aircraft engines are in development. These engines aim to reduce aviation emissions and explore alternative propulsion systems for cleaner air travel.
Nanotechnology Applications
Nanostructured Components: Nanotechnology is being used to develop nanostructured materials for various engine components, enhancing their efficiency, durability, and performance in hydrogen-powered systems.
These emerging engine designs and prototypes showcase the ongoing innovation and diversity in hydrogen engine technology. They hold the promise of cleaner and more efficient energy solutions across diverse sectors.
C. Fuel Cell Advancements
Enhanced Fuel Cell Performance: Ongoing research aims to improve fuel cell efficiency, durability, and cost-effectiveness. Advancements in materials and designs seek to enhance the performance of hydrogen fuel cells in various applications.
Solid-State Hydrogen Fuel Cells: Development of solid-state fuel cells that eliminate the need for liquid electrolytes could revolutionize hydrogen fuel cell technology, improving safety and efficiency.
D. Storage and Transportation
Advanced Storage Solutions: Innovations in materials science aim to develop novel storage materials capable of safely storing hydrogen at higher densities and lower pressures, improving the efficiency of hydrogen storage systems.
Hydrogen Carriers: Research investigates carrier materials or chemical compounds capable of safely transporting and releasing hydrogen, potentially circumventing some storage challenges.
E. Collaborations and Investments in Hydrogen Infrastructure
Hydrogen Refueling Infrastructure: Efforts are underway to expand hydrogen refuelling infrastructure globally. Innovations in station design, distribution networks, and cost-effective installation methods aim to promote the widespread adoption of hydrogen vehicles.
F. Cross-Sector Collaborations
Public-Private Partnerships: Collaborations between governments, research institutions, and private industries are fostering innovation. These partnerships accelerate research, development, and deployment of hydrogen technologies across sectors.
G. Renewable Integration
Hydrogen as Energy Storage: Research focuses on using hydrogen as a means to store surplus renewable energy, enabling better integration of intermittent renewable sources into the energy grid.
H. Safety and Standards
Standardization and Safety Protocols: Ongoing efforts aim to establish global standards and safety protocols for hydrogen production, storage, and usage, ensuring safe and standardized practices across industries.
These ongoing research initiatives and innovations underscore the dynamic nature of hydrogen engine technology. Continued investment and collaboration in these areas hold the potential to overcome existing challenges and drive the widespread adoption of hydrogen as a clean and sustainable energy solution.
8. Economic and Policy Implications:
The economic and policy implications surrounding hydrogen engine technology play a crucial role in its adoption and integration into the energy landscape. Here are the key aspects:
A. Economic Implications
Market Growth and Investment: The development and adoption of hydrogen engines represent significant economic opportunities. Investments in research, infrastructure, and manufacturing could stimulate economic growth, create jobs, and spur innovation in related industries.
Cost Competitiveness: Lowering production costs for hydrogen, fuel cells, and associated technologies is critical to making hydrogen engines economically competitive with traditional fossil fuel-based systems. Cost reduction measures will enhance market penetration and widespread adoption.
Industry Growth and Opportunities: The shift towards hydrogen-based technologies could lead to the growth of new industries and business opportunities. This includes manufacturing of hydrogen-related components, infrastructure development, and service sectors.
B. Policy Implications
Regulatory Frameworks: Establishing supportive regulatory frameworks and policies is crucial. Governments can encourage the adoption of hydrogen engines through incentives, subsidies, tax credits, and regulatory support to stimulate investment and research.
Emissions Reduction Targets: Hydrogen engines play a pivotal role in achieving climate change goals by reducing emissions. Policymakers may incentivize the adoption of hydrogen technologies to meet emission reduction targets and promote a transition to cleaner energy sources.
Standardization and Certification: Developing standardized protocols and certification procedures ensures safety, interoperability, and quality control in the production, storage, and usage of hydrogen, fostering market confidence and growth.
International Cooperation: Collaboration between nations in setting common standards, sharing technological advancements, and harmonizing regulations accelerates the global adoption of hydrogen engines.
C. Energy Security and Independence
Diversification of Energy Sources: Hydrogen engines contribute to diversifying the energy mix, reducing dependence on finite fossil fuels, and enhancing energy security.
Reducing Geopolitical Risks: Shifting towards hydrogen-based energy reduces reliance on energy imports, potentially mitigating geopolitical risks associated with traditional fuel sourcing.
D. Transition Challenges
Transition Period Considerations: Policymakers need to address challenges during the transition phase from conventional to hydrogen-based technologies. This includes managing infrastructure upgrades, workforce transitions, and ensuring a fair transition for affected industries.
Navigating these economic and policy implications requires a concerted effort from governments, industries, and stakeholders. Strategic policies that incentivize innovation, investment, and adoption of hydrogen engines while addressing economic challenges will play a crucial role in shaping the future energy landscape.
9. Environmental Sustainability:
A. Renewable Energy Integration
Utilizing Renewable Sources: Hydrogen can be produced using renewable energy sources like solar, wind, or hydroelectric power through electrolysis. This process creates “green hydrogen,” ensuring that the fuel’s production is sustainable and aligned with renewable energy objectives.
B. Reducing Fossil Fuel Dependency
Transitioning Away from Fossil Fuels: Hydrogen engines offer an alternative to traditional fossil fuel-based engines, reducing reliance on finite and environmentally harmful resources like oil and coal. This shift supports the transition to cleaner and more sustainable energy sources.
C. Carbon Footprint Reduction and Environmental Benefits: Zero Emissions:
Emission-Free Operation: Hydrogen engines produce zero greenhouse gas emissions during operation, emitting only water vapor as a byproduct. This characteristic significantly reduces air pollution and minimizes the carbon footprint, contributing to cleaner air quality and combating climate change.
D. Energy Efficiency:
Higher Efficiency: Hydrogen engines often boast higher efficiency compared to conventional combustion engines, translating into better fuel economy and reduced energy waste. This increased efficiency contributes to overall energy conservation.
E. Mitigating Climate Change:
Greenhouse Gas Reduction: By replacing conventional engines with hydrogen-powered ones, the overall emission of greenhouse gases, particularly carbon dioxide, is significantly reduced. This aids in mitigating climate change and its associated environmental impacts.
F. Environmental Restoration:
Promoting Ecosystem Restoration: As hydrogen engines reduce harmful emissions, they indirectly contribute to the preservation and restoration of ecosystems by curbing air and water pollution.
G. Sustainable Urban Environments:
Improving Urban Air Quality: Hydrogen engines in urban settings, particularly for public transportation, contribute to reducing air pollution, improving air quality, and creating healthier urban environments.
H. Circular Economy Approach:
Recycling and Reusing Resources: Hydrogen production often involves utilizing recycled water and materials, supporting a circular economy approach by minimizing waste and maximizing resource efficiency.
I. Sustainable Development Goals (SDGs):
Alignment with SDGs: The adoption of hydrogen engines aligns with various Sustainable Development Goals (SDGs) set by the United Nations, particularly in promoting clean energy, climate action, and sustainable cities and communities.
These environmental sustainability benefits position hydrogen engines as a promising and crucial component in achieving a more sustainable and environmentally friendly energy future.
10. Future Prospects and Forecasts:
The future prospects and forecasts for hydrogen engines depict a promising trajectory in the realm of clean energy. Here are the anticipated trends and prospects:
A. Accelerated Adoption:
Increased Integration: Forecasts suggest an increased adoption of hydrogen engines across various sectors, including transportation, power generation, and industrial applications, driven by growing environmental concerns and a push for sustainable energy solutions.
Expanded Applications: Anticipated expansion of hydrogen engine applications beyond vehicles, encompassing maritime transport, aviation, stationary power generation, and integration into various industries.
B. Technological Advancements:
Innovations and Efficiency: Forecasts predict continuous technological advancements in hydrogen engine technology, enhancing efficiency, reliability, and performance while reducing production costs.
Improved Infrastructure: Advancements in infrastructure development, including expanded refueling stations and optimized distribution networks, will support the wider adoption of hydrogen-powered vehicles and systems.
C. Renewable Integration
Increased Green Hydrogen Production: Forecasts indicate a rise in the production of “green hydrogen,” generated from renewable energy sources like solar and wind, fostering sustainable production practices.
Renewable Energy Synergies: Anticipated integration of hydrogen as an energy carrier in conjunction with increasing renewable energy sources, providing storage solutions and balancing grid demands.
D. Market Growth and Investment
Growing Market Opportunities: Forecasts suggest an expanding market for hydrogen-related technologies, creating opportunities for investments, job creation, and economic growth across various industries.
Economic Viability: Anticipated cost reductions and improved competitiveness of hydrogen engines will drive increased investments and market viability.
E. Policy Support and Global Collaboration
Supportive Policies: Forecasts anticipate governments implementing supportive policies, incentives, and regulations that encourage the adoption of hydrogen engines, fostering innovation and market growth.
International Collaboration: Predicted collaborations and agreements between nations to standardize regulations, share technological advancements, and develop a cohesive global framework for hydrogen technology.
F. Environmental Impact
Emission Reductions: Forecasts indicate significant reductions in greenhouse gas emissions through the widespread adoption of hydrogen engines, contributing to climate change mitigation efforts.
Air Quality Improvement: Anticipated improvements in urban air quality due to reduced emissions from hydrogen-powered vehicles and industrial applications.
The future prospects for hydrogen engines are promising, driven by technological advancements, supportive policies, growing market opportunities, and a global shift towards sustainable and clean energy solutions. These forecasts suggest a pivotal role for hydrogen engines in shaping a more sustainable and environmentally friendly energy landscape.
FAQ.
A hydrogen engine operates by using hydrogen gas as its primary fuel source. It works through a process called combustion, where hydrogen mixes with oxygen from the air to generate energy.
In a hydrogen engine, the hydrogen gas is typically stored in a tank and then delivered to the engine’s combustion chamber. When the hydrogen mixes with oxygen, either through an intake or directly injected into the chamber, it creates a highly combustible mixture.
When ignited, this mixture undergoes combustion, producing energy in the form of heat. This energy is then converted into mechanical energy, which powers the engine and drives the vehicle or machinery.
The key benefit of a hydrogen engine is that its only emission is water vapor, making it an environmentally friendly alternative to traditional combustion engines that produce greenhouse gases.
Aspect | Hydrogen Engines | Traditional Combustion Engines |
---|---|---|
Fuel Type | Hydrogen gas | Gasoline, Diesel |
Emissions | Water vapor (Zero greenhouse gas) | CO2, NOx, particulate matter |
Combustion Process | Hydrogen + Oxygen combustion | Fuel-air mixture combustion |
Efficiency | Potential for higher efficiency | Varies, generally lower |
Infrastructure | Developing, limited fueling stations | Established fueling stations |
Technology Status | Ongoing R&D, evolving technology | Mature, refined technology |
Widespread adoption of hydrogen technology faces several challenges:
Infrastructure: Building a comprehensive hydrogen infrastructure, including production, storage, and distribution facilities, is crucial. Establishing hydrogen refueling stations for vehicles or integrating hydrogen into existing energy grids requires substantial investment and planning.
Costs: Hydrogen production, especially using methods like electrolysis, can be expensive compared to conventional fuels. Reducing production costs and making hydrogen technologies more economically competitive is a significant hurdle.
Storage and Transportation: Storing and transporting hydrogen efficiently and safely remain challenges. Hydrogen has low energy density by volume, requiring advanced storage solutions to increase energy density and ensure safe handling.
Technology Development: Advancements are needed in fuel cell technology and hydrogen engines to improve efficiency, durability, and reliability while reducing manufacturing costs.
Regulatory Support: Policies and regulations that support the development of hydrogen infrastructure and incentivize adoption are crucial. This includes subsidies, tax incentives, emissions regulations, and standards for safety and performance.
Public Awareness and Acceptance: Educating the public about the benefits and safety of hydrogen technology is essential for its acceptance and adoption. Overcoming skepticism and increasing awareness about the potential of hydrogen as a clean energy source is vital.
Addressing these challenges requires collaboration among governments, industries, and research institutions to drive innovation, reduce costs, and create a supportive environment for the widespread adoption of hydrogen technology.